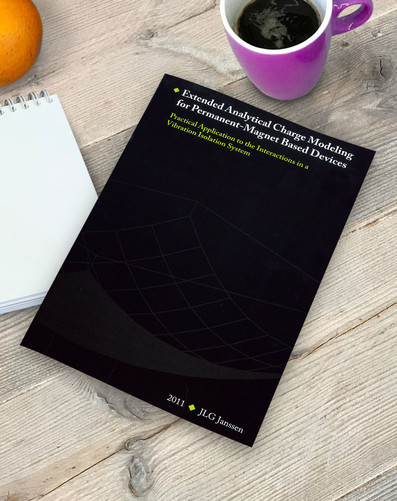
This thesis researches the analytical surface charge modeling technique which provides a fast, mesh-free and accurate description of complex unbound electromagnetic problems. To date, it has scarcely been used to design passive and active permanent-magnet devices, since ready-to-use equations were still limited to a few domain areas. Although publications available in the literature have demonstrated the surface-charge modeling potential, they have only scratched the surface of its application domain. The research that is presented in this thesis proposes ready-to-use novel analytical equations for force, stiffness and torque. The analytical force equations for cuboidal permanent magnets are now applicable to any magnetization vector combination and any relative position. Symbolically derived stiffness equations directly provide the analytical 3 £ 3 stiffness matrix solution. Furthermore, analytical torque equations are introduced that allow for an arbitrary reference point, hence a direct torque calculation on any assembly of cuboidal permanent magnets. Some topics, such as the analytical calculation of the force and torque for rotated magnets and extensions to the field description of unconventionally shaped magnets, are outside the scope of this thesis are recommended for further research. A worldwide first permanent-magnet-based, high-force and low-stiffness vibration isolation system has been researched and developed using this advanced modeling technique. This one-of-a-kind 6-DoF vibration isolation system consumes a minimal amount of energy (Ç 1W) and exploits its electromagnetic nature by maximizing the isolation bandwidth (> 700Hz). The resulting system has its resonance > 1Hz with a -2dB per decade acceleration slope. It behaves near-linear throughout its entire 6-DoF working range, which allows for uncomplicated control structures. Its position accuracy is around 4mum, which is in close proximity to the sensor’s theoretical noise level of 1mum. The extensively researched passive (no energy consumption) permanent-magnet based gravity compensator forms the magnetic heart of this vibration isolation system. It combines a 7.1kN vertical force with <10kN/m stiffness in all six degrees of freedom. These contradictory requirements are extremely challenging and require the extensive research into gravity compensator topologies that is presented in this thesis. The resulting cross-shaped topology with vertical airgaps has been filed as a European patent. Experiments have illustrated the influence of the ambient temperature on the magnetic behavior, 1.7h/K or 12N/K, respectively. The gravity compensator has two integrated voice coil actuators that are designed to exhibit a high force and low power consumption (a steepness of 625N2/W and a force constant of 31N/A) within the given current and voltage constraints. Three of these vibration isolators, each with a passive 6-DoF gravity compensator and integrated 2-DoF actuation, are able to stabilize the six degrees of freedom. The experimental results demonstrate the feasibility of passive magnet-based gravity compensation for an advanced, high-force vibration isolation system. Its modular topology enables an easy force and stiffness scaling. Overall, the research presented in this thesis shows the high potential of this new class of electromagnetic devices for vibration isolation purposes or other applications that are demanding in terms of force, stiffness and energy consumption. As for any new class of devices, there are still some topics that require further study before this design can be implemented in the next generation of vibration isolation systems. Examples of these topics are the tunability of the gravity compensator’s force and a reduction of magnetic flux leakage.
Download Thesis